Although they have been in the news over the last few weeks as a result of geopolitics, Rare Earth Minerals are much less well-known than they should be. This plural and general term does not correspond to their true nature, as they are really a set of 17 quite copious chemical elements that are very present in everyday life.
A trade conflict between China and the United States made this term an item for debate in the media, although up until then it had been practically unheard of outside specialized sectors. The news has revealed their present and future relevance, but what is really behind them? Rare Earth Elements are 17 chemical elements that are found in the Earth’s crust (15 make up the group of lanthanides, along with yttrium and scandium, which are included because they are frequently found in the same deposits). The lanthanides are: cerium, praseodymium, neodymium, promethium, samarium, europium, gadolinium, terbium, dysprosium, holmium, erbium, thulium, ytterbium, and lutetium.
Principal valor de los elementos
The main value of these elements is their exceptional magnetic, luminescent, and electrochemical properties. They are applied to a number of sectors and present in everyday objects including headphones, sensors, and goggles. A number of minerals (more than 180) are formed with these elements, and specifically lanthanides, except for promethium, are metal oxides in some 25 minerals, notably the highly used and financially profitable bastnäsite, monazite, xenotime, loparite, cerite, and gadolinite. Although they have been industrially mined over the last 50 years, they began to be isolated between the 18th and 19th centuries. “For example, promethium, one of the most rare, is a radioactive element that only exists for a few minutes and that was not isolated until 1945, pure lutetium metal was not refined until 1953, and yttrium was discovered in 1794,” explained Miguel Regueiro, president of the Official Spanish Geologists Association (ICOG).
Global production of these oxides is around 160,000 tons per year, and 95% come from China, which nevertheless has only 50% of the world’s reserves estimated at between 80 and 120 million tons, sufficient to cover demand for the next 625 years. Other forms of mining are also being researched, including one leveraging its presence in the crusts of cobalt magnesium.
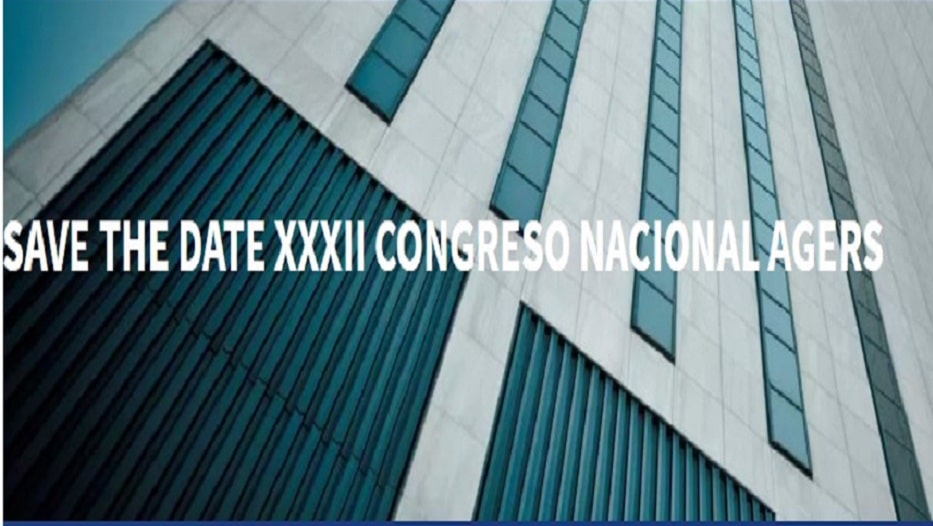
Global production of these oxides is around 160,000 tons per year, and 95% of the total comes from China.
Current commercial deposits of these minerals are mainly associated with four types of rocks:
1. Carbonatites. Mining for these rocks rich in carbonates is being carried out in deposits such as the Baya Obo (Mongolia) and Mountain Pass (United States) mines.
2. Alkaline igneous rocks. For example, the nepheline syenite deposit in Lovozero, Russia.
3. Laterite clays. Result of the alteration of the deposits mentioned above, especially mined in southeastern China.
4. Placer deposits, such as the Matamulas Monazite deposit in Ciudad Real, Spain.
Although they have still not been mined, Spain also has Rare Earth reserves, but production companies have not been able to start mining due to a number of problems and contextual impediments, even after operations are approved. According to Miguel Regueiro, Spain’s potential is undeniable, but the resources and willpower necessary to understand their scope are not being put out. “These resources have barely been researched, so it would be a bit much to say that we have sufficient resources. Nevertheless, there are indications that those resources exist and even a few deposits, such as Matamulas in Ciudad Real and Monte Galiñeiro in Pontevedra, have been assessed,” he said.
Prospecting and risks
Beginning the mining process usually involves carrying out a number of phases that can take more than 15 years. The approximate timeline for developing conventional projects is as follows:
– Resource exploration (4 to 6 years)
– Context, scope, and pilot plant (at least 6 years)
– Funding (at least 3 years)
– Optimized launch (1 year)
Subsequent processing depends on the oxide of the Rare Earths mined, although it is usually considered a complex process. Throughout all of these phases, prior to industrial use, certain risks arising from the activity are faced. “All mining projects have environmental impacts,” explained the geologist, “so the law is highly restrictive with regard to the environmental impact of projects and the obligation to restore the land affected once the operation has been completed.” Regueiro nevertheless ensured that if said projects are carried out in full compliance with current regulations, their impact is limited and eventually offset and the land is restored to its initial state without major issues. “Often times areas affected by mining operations become areas environmentally much better off than they were before the operation took place,” he said. “Each case and process also involves different environmental impacts. For example, in the only case studied in Spain (the Matamulas deposit) consisting of gravel with monazite, the most significant environmental claims are the planned amount of water consumption and potential alterations to the flora and fauna, which was the reason why said project has not yet been authorized.”
Processing risks, however, are similar to those of other industrial installations and therefore they must also comply with common conditions.
Properties and applications
The electron configuration of Rare Earth Elements is the key to the physical and chemical properties that are of use to industry: catalysts, electronics, magnets, optics, glass, ceramics, and metallurgy. The elements obtained from the minerals have an infinite number of industrial applications.
“Samarium, for example, is used in strong permanent magnets that allow for the development of modern electric engines. The storage of computer data that allows devices to be smaller and have greater capacity owes some of its advancement to the extraordinary magnetic properties of ytterbium and terbium. And the fantastic reds on flat screens, which replaced old cathode-ray tubes, are highly related to europium and yttrium,” said the president of the ICOG.
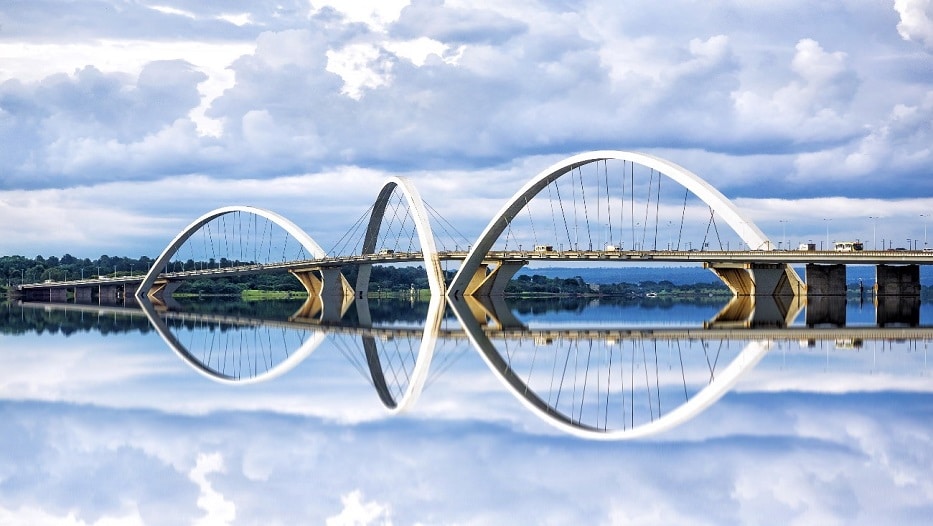
Neodymium is an element essential to the manufacture of electric cars and it is therefore key to the future of the automotive industry.
Neodymium is one of the elements with the most applications on the market. It is used, for example, in the development of tempered glass with a ceramic glaze stain that is able to optimize resistance, a significant advancement in the manufacturing of goggles used by welders, as it absorbs the amber light of the voltage arc.
Its tone also provides delicate colors to other kinds of glass that are used in basic technological products for sectors such as astrophysics (astronomers use them to calibrate their spectrometers and infrared radiation filters) and tech, as they are the basis for high-intensity field magnets. These inexpensive and strong magnets are commonly used in the manufacture of headphones, speakers, hard drives, and sensors.
Beyond these specific cases, Rare Earth Elements are used in many more contexts such as oil refining processes, cooling, energy storage, specialized glass, nuclear batteries and x-ray tubes. They are also present in an infinite number of everyday objects such as telephones, fluorescent lights, and cameras. One of the industries that has used these 17 elements the most is the auto industry, as they are essential for the manufacture of electric vehicles. Their engines use magnets made of materials such as dysprosium, gadolinium, and neodymium, while cerium is part of catalytic converters and hybrid batteries.
Although they are sold by the kilo – and their price varies from 1.70 dollars per kilo of cerium to 730 dollars per kilo of terbium – they are added by the gram to products. The immense reserves and versatility of these elements promise a great future, although Manuel Regueiro ensured that, despite the trend indicating certain sectors as those that will benefit the most from their properties, nothing should be taken for granted. “It is hard to predict,” he warned, “but the trend of decarbonization and electrification of global transport, catalytic converters, batteries, and wind turbines will surely be the fields that see the most growth in the use of these elements. In any case, the key lies their extraordinary physical and chemical properties.”
This article was written with assistance from…
Manuel Regueiro y González-Barros holds a bachelor’s degree in Geology from the Complutense University of Madrid and a master’s degree in Leadership and Public Management from Menéndez Pelayo International University. After working 17 years as an industrial rock and mineral specialist at the Geology and Mineral Resource Institute of Spain, in 2005 he was offered the position of head of the External Relations and Communications Area. From 1992 to 2013 he was an adjunct professor in the Crystallography and Mineralogy at UCM, where he is now an honorary collaborator.
He was elected president of the European Federation of Geologists on two non-consecutive occasions, has been a member, general secretary and first vice chairman of the Official Spanish Geologists Association, where he was elected chairman in 2016, and was a founding member and secretary of the NGO Geólogos del Mundo. In 2017 he was elected vice chairman of the Professional Union.
Author and coauthor of 14 books, over 105 articles in specialized journals and presenter of more than 160 talks at conferences and seminars, he just released his latest popular science book, Para qué sirve la geología [What is geology for?], written with his daughter Macarena and published jointly by the Geology and Mineral Resource Institute of Spain and the publishing house La Catarata.
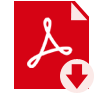